Source: hearthmath.com
------------------------------
Many of the changes in bodily function that occur during the coherence state revolve around changes in the heart’s pattern of activity. While the heart is certainly a remarkable pump, interestingly, it is only relatively recently in the course of human history—around the past three centuries or so—that the heart’s function has been defined (by Western scientific thought) as only that of pumping blood. Historically, in almost every culture of the world, the heart was ascribed a far more multifaceted role in the human system, being regarded as a source of wisdom, spiritual insight, thought, and emotion. Intriguingly, scientific research over the past several decades has begun to provide evidence that many of these long-surviving associations may well be more than simply metaphorical. These developments have led science to once again to revise and expand its understanding of the heart and the role of this amazing organ.
In the new field of neurocardiology, for example, scientists have discovered that the heart possesses its own intrinsic nervous system—a network of nerves so functionally sophisticated as to earn the description of a “heart brain.” Containing over 40,000 neurons, this “little brain” gives the heart the ability to independently sense, process information, make decisions, and even to demonstrate a type of learning and memory. In essence, it appears that the heart is truly an intelligent system. Research has also revealed that the heart is a hormonal gland, manufacturing and secreting numerous hormones and neurotransmitters that profoundly affect brain and body function. Among the hormones the heart produces is oxytocin—well known as the “love” or “bonding hormone.” Science has only begun to understand the effects of the electromagnetic fields produced by the heart, but there is evidence that the information contained in the heart’s powerful field may play a vital synchronizing role in the human body—and that it may affect others around us as well.
Research has also shown that the heart is a key component of the emotional system. Scientists now understand that the heart not only responds to emotion, but that the signals generated by its rhythmic activity actually play a major part in determining the quality of our emotional experience from moment to moment. As described next, these heart signals also profoundly impact perception and cognitive function by virtue of the heart’s extensive communication network with the brain. Finally, rigorous electrophysiological studies conducted at the HeartMath Institute have even indicated that the heart appears to play a key role in intuition. Although there is much yet to be understood, it appears that the age-old associations of the heart with thought, feeling, and insight may indeed have a basis in science.
The Heart–Brain Connection
Most of us have been taught in school that the heart is constantly responding to “orders” sent by the brain in the form of neural signals. However, it is not as commonly known that the heart actually sends more signals to the brain than the brain sends to the heart! Moreover, these heart signals have a significant effect on brain function – influencing emotional processing as well as higher cognitive faculties such as attention, perception, memory, and problem-solving. In other words, not only does the heart respond to the brain, but the brain continuously responds to the heart.
The effect of heart activity on brain function has been researched extensively over about the past 40 years. Earlier research mainly examined the effects of heart activity occurring on a very short time scale – over several consecutive heartbeats at maximum. Scientists at the HeartMath Institute have extended this body of scientific research by looking at how larger-scale patterns of heart activity affect the brain’s functioning.
HeartMath research has demonstrated that different patterns of heart activity (which accompany different emotional states) have distinct effects on cognitive and emotional function. During stress and negative emotions, when the heart rhythm pattern is erratic and disordered, the corresponding pattern of neural signals traveling from the heart to the brain inhibits higher cognitive functions. This limits our ability to think clearly, remember, learn, reason, and make effective decisions. (This helps explain why we may often act impulsively and unwisely when we’re under stress.) The heart’s input to the brain during stressful or negative emotions also has a profound effect on the brain’s emotional processes—actually serving to reinforce the emotional experience of stress.
In contrast, the more ordered and stable pattern of the heart’s input to the brain during positive emotional states has the opposite effect – it facilitates cognitive function and reinforces positive feelings and emotional stability. This means that learning to generate increased heart rhythm coherence, by sustaining positive emotions, not only benefits the entire body, but also profoundly affects how we perceive, think, feel, and perform.
Your Heart’s Changing Rhythm
The heart at rest was once thought to operate much like a metronome, faithfully beating out a regular, steady rhythm. Scientists and physicians now know, however, that this is far from the case. Rather than being monotonously regular, the rhythm of a healthy heart-even under resting conditions – is actually surprisingly irregular, with the time interval between consecutive heartbeats constantly changing. This naturally occurring beat-to-beat variation in heart rate is called heart rate variability (HRV).
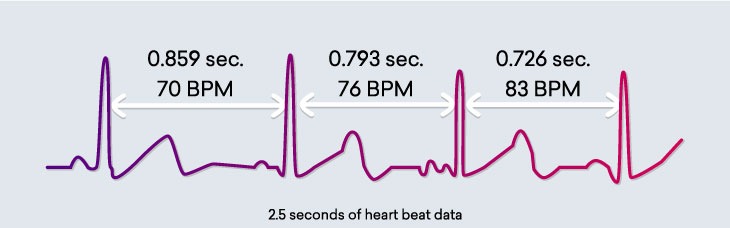
Heart rate variability is a measure of the beat-to-beat changes in heart rate. This diagram shows three heartbeats recorded on an electrocardiogram (ECG). Note that variation in the time interval between consecutive heartbeats, giving a different heart rate (in beats per minute) for each interbeat interval.
The normal variability in heart rate is due to the synergistic action of the two branches of the autonomic nervous system (ANS)—the part of the nervous system that regulates most of the body’s internal functions. The sympathetic nerves act to accelerate heart rate, while the parasympathetic (vagus) nerves slow it down. The sympathetic and parasympathetic branches of the ANS are continually interacting to maintain cardiovascular activity in its optimal range and to permit appropriate reactions to changing external and internal conditions. The analysis of HRV therefore serves as a dynamic window into the function and balance of the autonomic nervous system.
The moment-to-moment variations in heart rate are generally overlooked when average heart rate is measured (for example, when your doctor takes your pulse over a certain period of time and calculates that your heart is beating at, say, 70 beats per minute). However, the emWave and Inner Balance technologies allows you to observe your heart’s changing rhythms in real time. Using your pulse data, it provides a picture of your HRV—plotting the natural increases and decreases in your heart rate occurring on a continual basis.
Why is HRV Important?
Scientists and physicians consider HRV to be an important indicator of health and fitness. As a marker of physiological resilience and behavioral flexibility, it reflects our ability to adapt effectively to stress and environmental demands. A simple analogy helps to illustrate this point: just as the shifting stance of a tennis player about to receive a serve may facilitate swift adaptation, in healthy individuals the heart remains similarly responsive and resilient, primed and ready to react when needed.
HRV is also a marker of biological aging. Our heart rate variability is greatest when we are young, and as we age the range of variation in our resting heart rate becomes smaller. Although the age-related decline in HRV is a natural process, having abnormally low HRV for one’s age group is associated with increased risk of future health problems and premature mortality. Low HRV is also observed in individuals with a wide range of diseases and disorders. By reducing stress-induced wear and tear on the nervous system and facilitating the body’s natural regenerative processes, regular practice of HeartMath coherence-building techniques can help restore low HRV to healthy values.
Heart Rhythm Patterns and Emotions
Many factors affect the activity of the ANS, and therefore influence HRV. These include our breathing patterns, physical exercise, and even our thoughts. Research at the HeartMath Institute has shown that one of the most powerful factors that affect our heart’s changing rhythm is our feelings and emotions. When our varying heart rate is plotted over time, the overall shape of the waveform produced is called the heart rhythm pattern. When you use the emWave and Inner Balance technologies, you are seeing your heart rhythm pattern in real time. HeartMath research has found that the emotions we experience directly affect our heart rhythm pattern – and this, in turn, tells us much about how our body is functioning.
In general, emotional stress – including emotions such as anger, frustration, and anxiety—gives rise to heart rhythm patterns that appear irregular and erratic: the HRV waveform looks like a series of uneven, jagged peaks (an example is shown in the figure below). Scientists call this an incoherent heart rhythm pattern. Physiologically, this pattern indicates that the signals produced by the two branches of the ANS are out of sync with each other. This can be likened to driving a car with one foot on the gas pedal (the sympathetic nervous system) and the other on the brake (the parasympathetic nervous system) at the same time – this creates a jerky ride, burns more gas, and isn’t great for your car, either! Likewise, the incoherent patterns of physiological activity associated with stressful emotions can cause our body to operate inefficiently, deplete our energy, and produce extra wear and tear on our whole system. This is especially true if stress and negative emotions are prolonged or experienced often.
In contrast, positive emotions send a very different signal throughout our body. When we experience uplifting emotions such as appreciation, joy, care, and love; our heart rhythm pattern becomes highly ordered, looking like a smooth, harmonious wave (an example is shown in the figure below). This is called a coherent heart rhythm pattern. When we are generating a coherent heart rhythm, the activity in the two branches of the ANS is synchronized and the body’s systems operate with increased efficiency and harmony. It’s no wonder that positive emotions feel so good – they actually help our body’s systems synchronize and work better.
Heart rhythm patterns during different emotional states. These graphs show examples of real-time heart rate variability patterns (heart rhythms) recorded from individuals experiencing different emotions. The incoherent heart rhythm pattern shown in the top graph, characterized by its irregular, jagged waveform, is typical of stress and negative emotions such as anger, frustration, and anxiety. The bottom graph shows an example of the coherent heart rhythm pattern that is typically observed when an individual is experiencing a sustained positive emotion, such as appreciation, compassion, or love. The coherent pattern is characterized by its regular, sine-wave-like waveform. It is interesting to note that the overall amount of heart rate variability is actually the same in the two recordings shown below; however, the patterns of the HRV waveforms are clearly different.
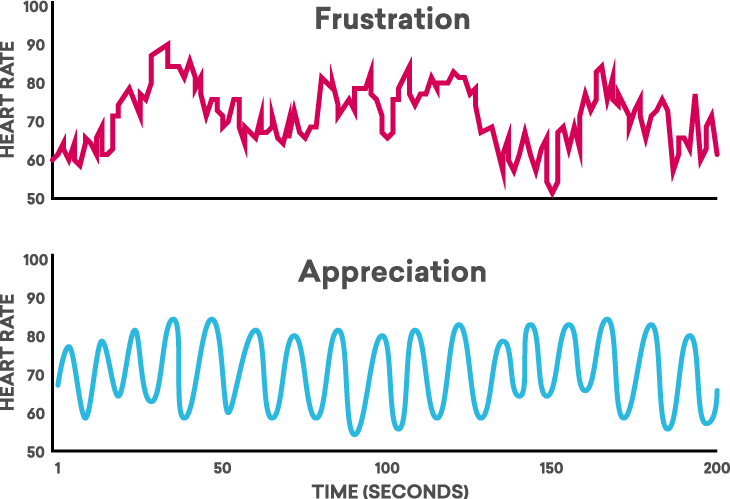
Coherence: A State of Optimal Function
The HeartMath Institute’s research has shown that generating sustained positive emotions facilitates a body-wide shift to a specific, scientifically measurable state. This state is termed psychophysiological coherence, because it is characterized by increased order and harmony in both our psychological (mental and emotional) and physiological (bodily) processes. Psychophysiological coherence is state of optimal function. Research shows that when we activate this state, our physiological systems function more efficiently, we experience greater emotional stability, and we also have increased mental clarity and improved cognitive function. Simply stated, our body and brain work better, we feel better, and we perform better.
Physiologically, the coherence state is marked by the development of a smooth, sine-wave-like pattern in the heart rate variability trace. This characteristic pattern, called heart rhythm coherence, is the primary indicator of the psychophysiological coherence state, and is what the emWave and Inner Balance technologies measure and quantify. A number of important physiological changes occur during coherence. The two branches of the ANS synchronize with one another, and there is an overall shift in autonomic balance toward increased parasympathetic activity. There is also increased physiological entrainment—a number of different bodily systems synchronize to the rhythm generated by the heart (see figure below). Finally, there is increased synchronization between the activity of the heart and brain.
Physiological entrainment during coherence.
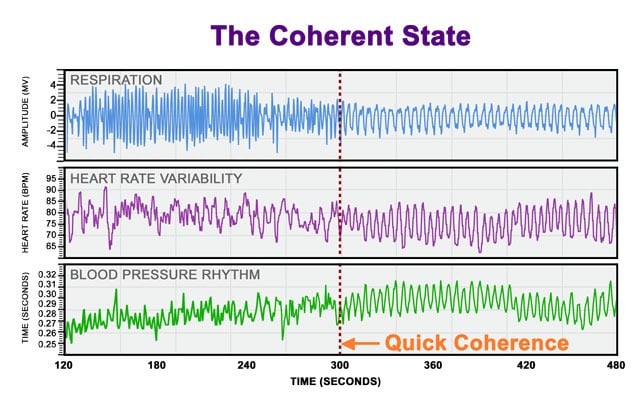
Coherence Is Not Relaxation
An important point is that the state of coherence is both psychologically and physiologically distinct from the state achieved through most techniques for relaxation. At the physiological level, relaxation is characterized by an overall reduction in autonomic outflow (resulting in lower HRV) and a shift in ANS balance towards increased parasympathetic activity. Coherence is also associated with a relative increase in parasympathetic activity, thus encompassing a key element of the relaxation response, but is physiologically distinct from relaxation in that the system oscillates at its natural resonant frequency and there is increased harmony and synchronization in nervous system and heart–brain dynamics. This important difference between the two states is reflected most clearly in their respective HRV power spectra (see figure and explanation below). Furthermore, unlike relaxation, the coherence state does not necessarily involve a lowering of heart rate, or a change in the amount of HRV, but rather is primarily marked by a change in the heart rhythm pattern.
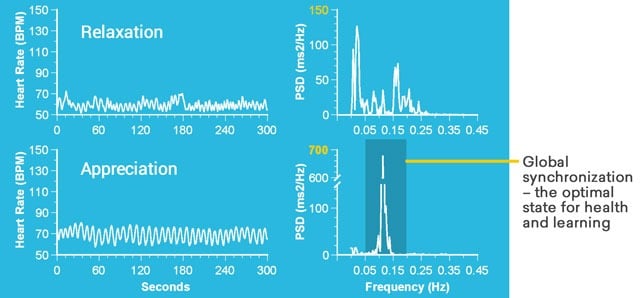
Unlike relaxation, coherence does not necessarily involve a reduction in HRV, and may at times even produce an increase in HRV relative to a baseline state. As can be seen in the corresponding power spectrum, coherence is marked by an unusually large, narrow peak in the low frequency band, centered around 0.1 hertz (note the significant power scale difference between the spectra for coherence and relaxation). This large, characteristic spectral peak is indicative of the system-wide resonance and synchronization that occurs during the coherence state.
Not only are there fundamental physiological differences between relaxation and coherence, but the psychological characteristics of these states are also quite different. Relaxation is a low-energy state in which the individual rests both the body and mind, typically disengaging from cognitive and emotional processes. In contrast, coherence generally involves the active engagement of positive emotions. Psychologically, coherence is experienced as a calm, balanced, yet energized and responsive state that is conducive to everyday functioning and interaction, including the performance of tasks requiring mental acuity, focus, problem-solving, and decision-making, as well as physical activity and coordination.
The Role of Breathing
Another important distinction involves understanding the role of breathing in the generation of coherence and its relationship to the techniques of the HeartMath System. Because breathing patterns modulate the heart’s rhythm, it is possible to generate a coherent heart rhythm simply by breathing slowly and regularly at a 10-second rhythm (5 seconds on the in-breath and 5 seconds on the out-breath). Breathing rhythmically in this fashion can thus be a useful intervention to initiate a shift out of stressful emotional state and into increased coherence. However, this type of cognitively-directed paced breathing can require considerable mental effort and is difficult for some people to maintain.
While HeartMath techniques incorporate a breathing element, paced breathing is not their primary focus and they should therefore not be thought of simply as breathing exercises. The main difference between the HeartMath tools and most commonly practiced breathing techniques is the HeartMath tools’ focus on the intentional generation of a heartfelt positive emotional state. This emotional shift is a key element of the techniques’ effectiveness. Positive emotions appear to excite the system at its natural resonant frequency and thus enable coherence to emerge and to be maintained naturally, without conscious mental focus on one’s breathing rhythm.
This is because input generated by the heart’s rhythmic activity is actually one of the main factors that affect our breathing rate and patterns. When the heart’s rhythm shifts into coherence as a result of a positive emotional shift, our breathing rhythm automatically synchronizes with the heart, thereby reinforcing and stabilizing the shift to system-wide coherence.
Additionally, the positive emotional focus of the HeartMath techniques confers a much wider array of benefits than those typically achieved through breathing alone. These include deeper perceptual and emotional changes, increased access to intuition and creativity, cognitive and performance improvements, and favorable changes in hormonal balance.
To derive the full benefits of the HeartMath tools, it is therefore important to learn how to self-activate and eventually sustain a positive emotion. However, for users who initially have trouble achieving or maintaining coherence, practicing heart-focused breathing at a 10-second rhythm, as described above, can be useful training aid. Once individuals grow accustomed to generating coherence through rhythmic breathing and become familiar with how this state feels, they can then begin to practice breathing a positive feeling or attitude through the heart area in order to enhance their experience of the HeartMath tools and their benefits. Eventually, with continuity of practice, most people become able to shift into coherence by directly activating a positive emotion.